«- back to print version table of contents
Bow Echo and Strong Rear Inflow Jet
Detection
This signature is based on organisation on the mesoscale. A Bow Echo is a type of linear multicell characterised as a bow-shaped (or concave-shaped), 20-120 km line of convective cells. Bow Echoes can be either in the form of an isolated line or as a bowing segment within a meso-convective system (e.g., a squall line).
Use the radio buttons or click the image to switch between reflectivity and velocity imagery:
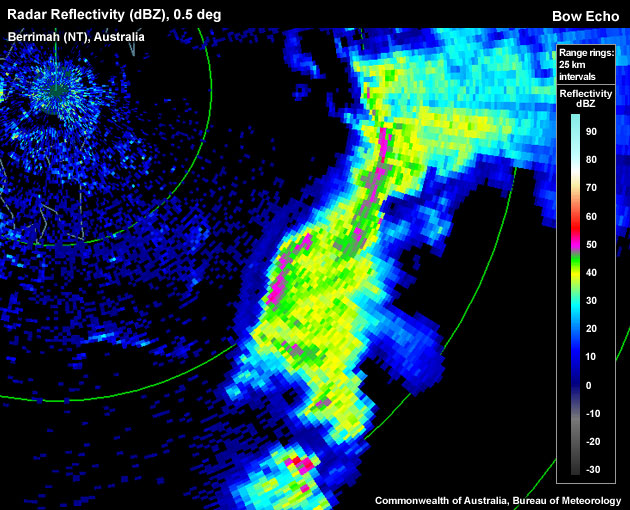
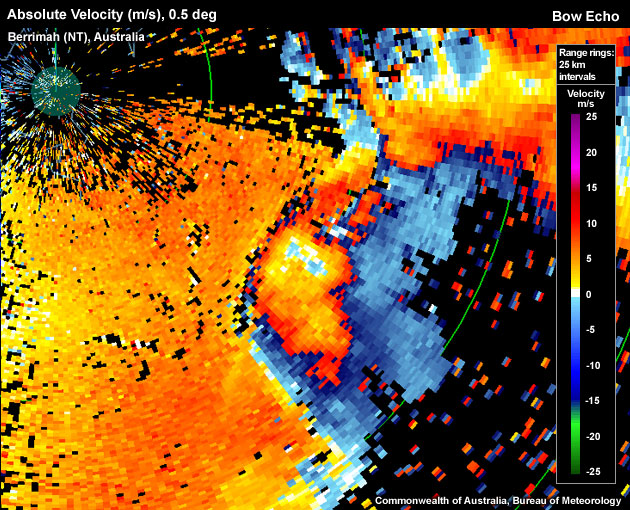
Bow Echo approaching Darwin (Australia) from the southeast; a gust front is apparent just ahead of the bow, and a Rear Inflow Notch (RIN) behind the apex of the bow.
One of the recognisable features of a Bow Echo is a Rear Inflow Notch (RIN). A RIN is a channel of weak reflectivties located well behind the core of the bow and it can signify the location of a strong Rear Inflow Jet (RIJ). A RIN is an especially useful feature for forecasters who have no access to Doppler data.
A RIJ is a velocity signature commonly found with a Bow Echo. A RIJ is an elevated jet accelerating (strictly, in a storm-relative frame of reference) from the rear to the front edge of the line of convective cells while descending towards the surface (and reaching it on occasion).
To determine if the signature you are seeing is a Bow Echo with a strong Rear Inflow Jet use the following criteria.
Reflectivity: PPI/Plan
- View using one of the lowest elevations; look for a continuous or broken up line of convective cells.
- Is the line of cells bowed in shape or are there any bowed 20-120 km long segments along the line? If so, a Bow Echo may be present.
- Supporting evidence for the identification of mesoscale convection as a Bow Echo is provided by the rear-inflow notch (RIN): on the “back” side of the bowing line (“back” with regards to the system's motion vector) a patch of weaker reflectivity might be apparent (the stratiform rain shield). Is there a notch of low or no reflectivity returns on the back side of that patch?
Velocity: PPI/Plan View
Here we are looking for a strong RIJ to provide additional support for the identification of a Bow Echo signature.
- In the vicinity of the bow's apex and the RIN (if present), look for a local maximum of velocity just behind the main line of convection. The velocity should decrease the further back you go from the leading edge of the line. Note: depending on the radar's Nyquits velocity the RIJ might be aliased in the velocity channel.
- Is the velocity maximum observed reaching or exceeding 48 knots? If so, a strong RIJ is present (damaging winds that have the potential of reaching the surface).
Velocity: RHI/Cross Section
An alternative view of the strong RIJ.
- Choose a cross section perpendicular to the line of convection, through the apex of the bowing segment.
- Is there evidence of a strong RIJ sloping down from rear to the front of the line of convection? If so, a strong RIJ is present.
Potential Difficulties in Detection
Radar sampling:
- Erroneous data, especially affects velocity data – Confidence in pixels is lowered.
- Resolution of the velocity data for the radar–Low radar resolution generally averages out extreme values.
- Resolution degradation as range increases – Radar sampling degrades as averaging becomes more enhanced due to a beam width.
- The thunderstorm is too far away from the radar – The radar overshoots the lower parts of the Bow Echo and the descending RIJ.
- Viewing angle of the radar – When trying to detect the strong RIJ in the velocity field, it may be difficult if the signature is not aligned with the radar beam as Doppler velocities only measure the radial velocity component of the wind field. A RIJ oriented perfectly across the radar beam is almost invisible.
- Storm-relative velocity – Ideally, the RIJ is viewed in a system-relative frame of reference by using system-relative Doppler velocities.
Examples of Bow Echo and Strong Rear Inflow Jet Signatures
Use the radio buttons or click the image to switch between reflectivity and velocity imagery:
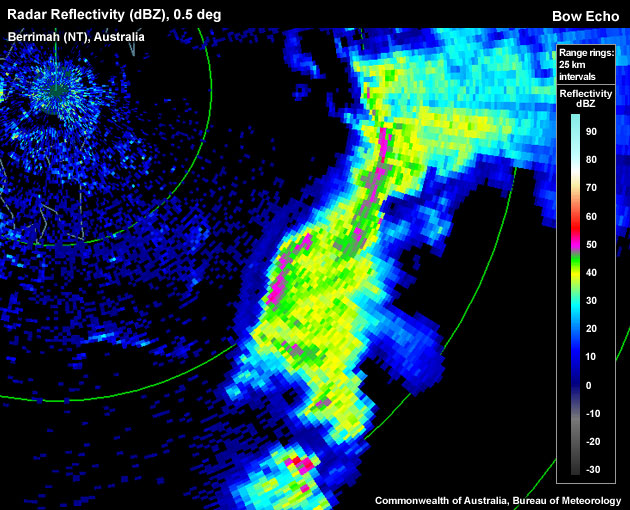
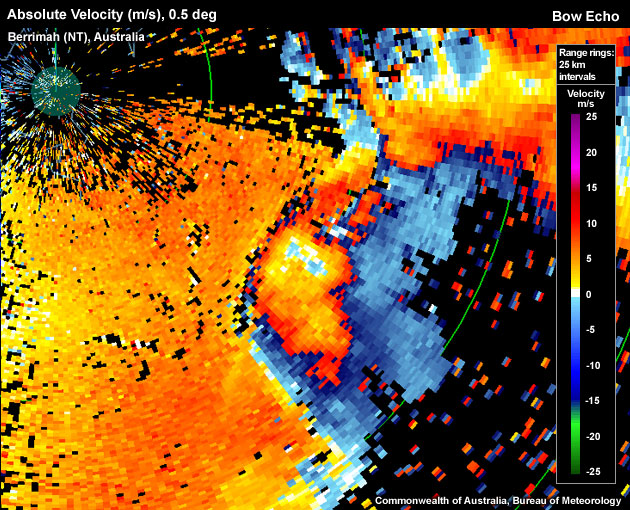
Bow Echo approaching Darwin (Australia) from the southeast; a gust front is apparent just ahead of the bow, and a Rear Inflow Notch (RIN) behind the apex of the bow.
Use the radio buttons or click the image to switch between reflectivity and velocity imagery:
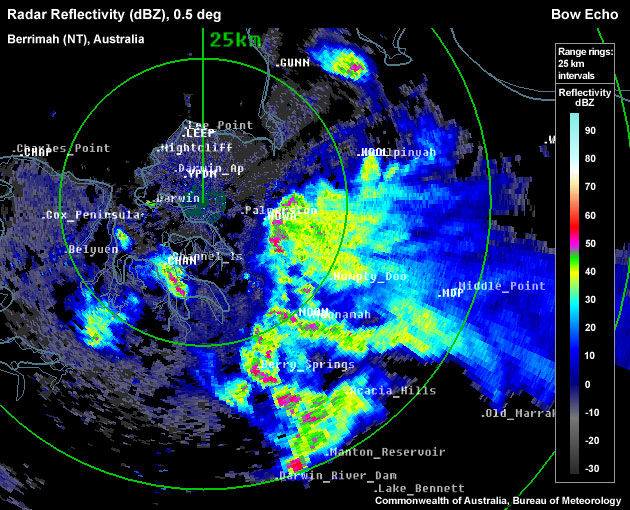
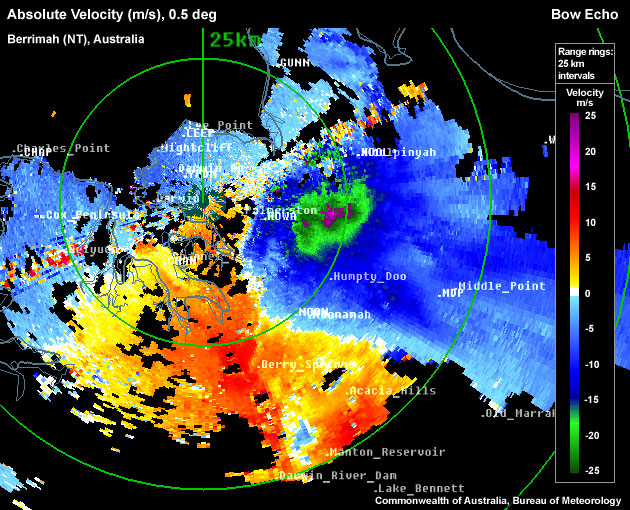
Bow Echo approaching Darwin (Australia) from the east; gust front out ahead, Rear Inflow Notch (RIN) behind the apex of the bow. Aliased Rear Inflow Jet (RIJ) is collocated with the RIN in velocity field.
Use the radio buttons or click the image to switch between reflectivity and velocity imagery:
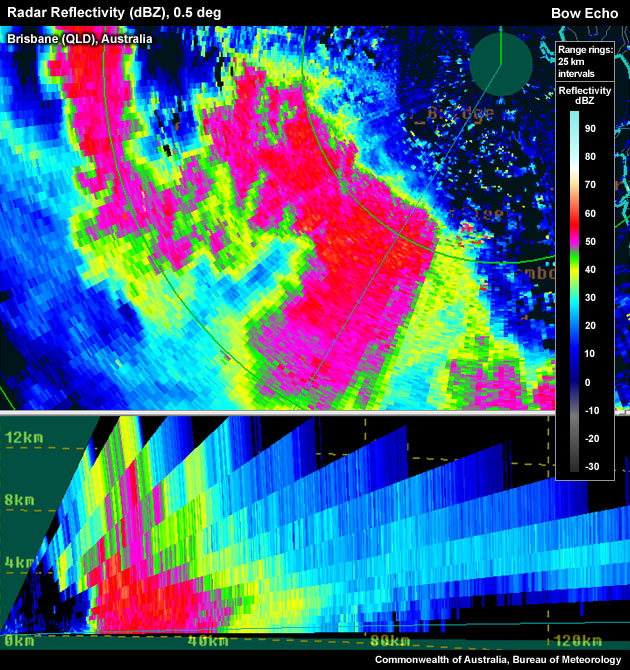
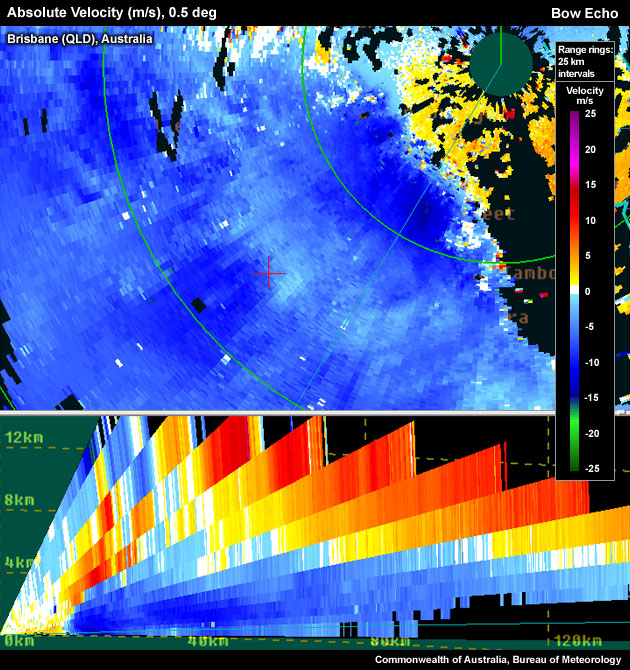
Example of a Bow Echo showing cross sections of the radar reflectivity and absolute velocity.
Conceptual Model
A Bow Echo signature can be used as one of many signatures pointing towards thunderstorm severity. The presence of a persistent Bow Echo signature should be associated with the presence of a strong Rear Inflow Jet (RIJ) which, if it descends to the surface, is likely to be associated with damaging winds.
RIJs are formed starting with a linear convective mode with warm air ascending toward the rear of the line into the anvil via leading edge updrafts (see figure below). Latent heat is released from this rising front to rear airstream forming a hydrostatic low beneath the anvil but above the cold pool. The cold pool marks a hydrostatically induced mesoscale high. Air flows laterally from both the rear and front of the line, into the low. The presence of a front-to-rear horizontal mesoscale pressure gradient above the system cold pool contributes to the rear-to-front acceleration of air parcels into the low near the leading edge of the system. Diabatic cooling of this rear inflow jet under the stratiform rain canopy contributes to its descent as it approaches the front of the system.
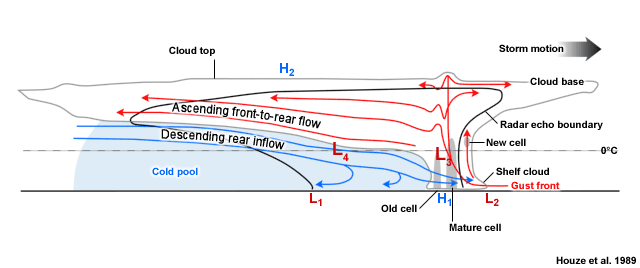
Schematic of a mature squall line (also representative of the central section of a Bow Echo) with a trailing stratiform precipitation region. The thick line indicates the approximate system outline on radar, the light scalloped line the approximate visual extent of the clouds. Stippled and solid fill areas mark regions of higher radar reflectivities.
This conceptual vertical cross-section of a trailing stratiform Mesoscale Convective System (MCS) is adapted from Houze et al (1989). More details can be found in MCS Storm Conceptual Models, Definition, Fig. 4.
Alongside the RIJ, Bow Echoes are characterised by the existence of cyclonic (poleward) and anticyclonic (equatorward) bookend vortices. In longer-lived Bow Echoes, the Coriolis force eventually favours the stronger development of the poleward vortex. The bow's apex is a preferred region for damaging surface winds, but small-scale mesovortices at the leading edge of the line can cause localised damage, in particular towards the poleward side of the apex.
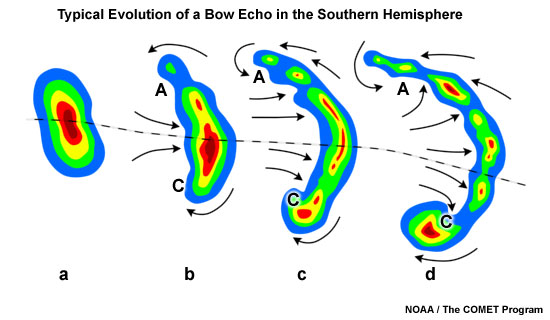
Typical evolution of a thunderstorm radar echo (a) in to a Bow Echo (b, c) and into a comma echo (d). Dashed line indicates axis of greatest potential for downbursts. Arrows indicate wind flow relative to the storm. Note regions of cyclonic rotation (C) and anticyclonic rotation (A); both regions, especially C, are capable of supporting tornado development in some cases.
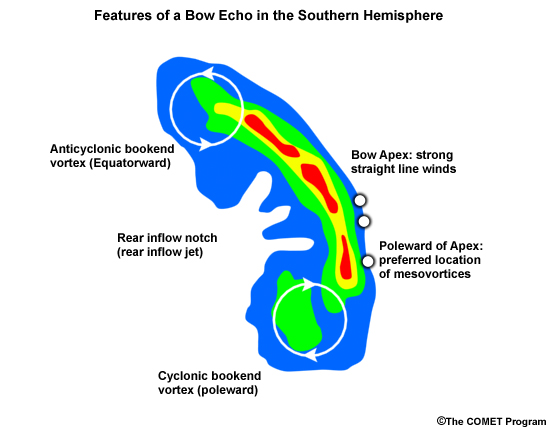
Schematic of a Bow Echo in the southern hemisphere. Reflectivity contours are shown, with the green fill colour denoting reflectivity values in the 30s (dBZ), and red colours in the 50s (dBZ). The apex region along the leading edge of the Bow Echo is often an area of damaging surface winds. The northern and southern ends of the Bow Echo are usually chracterised by bookend vortices. Damaging mesovortices, if they occur, are preferentially found along the leading edge of the Bow Echo, poleward of the apex location.
There is a noteworthy alternative viewpoint explaining the kinematic and dynamic properties of Bow Echoes: Weisman (1992) conceptualised the formation of the RIJ through the interaction of horizontal vorticity centres of opposite signs near the back of the stratiform rain shield and system cold pool.
Bow Echoes can either be an entity in itself or a smaller segment of a squall line. The bowed segment develops due to the potentially strong Rear Inflow Jet contributing significantly to a forward surge of the system outflow and associated bowing of the leading convective line.
Bow Echoes and strong Rear Inflow Jets are most likely to produce damaging winds, a criterion used to define a severe thunderstorm in Australia.
Bow Echoes are mostly made up of ordinary, non-rotating or weakly rotating cells, but they can contain supercells. Such embedded supercells are not as long-lived as more isolated supercells, as their inflow tends to be disrupted by other nearby cells within the line. Supercells tend to favour the ends of the line for development due to better access to unmodified low-level inflow. If a supercell is detected within a Bow Echo, an increased potential exists for all four severe convective hazards occurring: damaging or destructive winds, large or giant hail, heavy rainfall resulting in flash flooding
Determining Thunderstorm Classification
A significant Bow Echo with a strong Rear Inflow Jet is only associated with one type of thunderstorm classification: the Mesoscale Convective System (MCS).
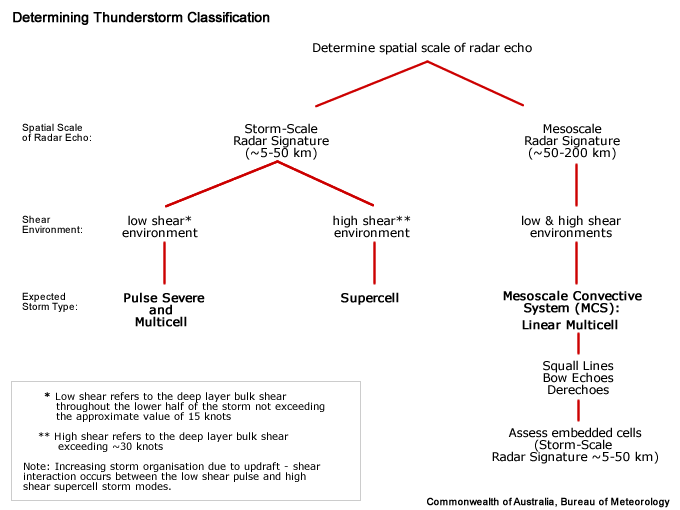
See Mesoscale Convective System (MCS) for more information.
Diagnosis
Once you have confidently identified a Bow Echo signature, this section will help you estimate the storm severity associated with it. Generally, the spatial and temporal scales of a signature are loosely related to the updraft strength. In other words, the larger and/or more long–lived the signature, the stronger the updraft that produced it. In velocity-based signatures, updraft severity can usually also be gauged by the magnitude of the measured radial velocities. Examining a storm's overall temporal evolution will suggest whether the storm is becoming more or less severe. Radar signatures and associated storm developments can also be time-shifted relative to each other, as is the case in supercell tornadoes that occur during the collapse of the parent storm.
When comparing signatures to diagnose relative severity, keep in mind that it is assumed that signatures are sampled at equal ranges from the radar. Otherwise, a storm sampled at greater range (with a wider beam) can appear to be weak and/or weakening, while a storm sampled at a closer range (with a narrower beam) can appear to be strong and/or strengthening.
Degree of Severity
The probability of damaging or destructive surface winds increases with:
- The magnitude of the radial velocity within the RIJ
- A decrease in height above ground level of the RIJ – as discussed in the Detection section, the lower the height of the damaging radial winds, the more likely it is that most of that momentum will be transferred to the surface increasing the likelihood of damaging surface winds.
- A decrease in static stability between the ground and the elevated RIJ – strong, pre-existing cold pools near the surface make it less likely that the RIJ can transport strong winds to the surface.
- Significant individual storm-scale severe signatures within the Bow Echo – as the Bow Echo is a Mesoscale signature it can possess any of the storm scale signatures, such as:
- WER
- BWER
- Mesocyclones
- 50 dBz Echo Top Height
- Storm Top Divergence
- TBSS
- Forward Flank Notch
- Tight Low Level Reflectivity Gradient
- Hook Echo
- Low Level Convergence
- Low Level Divergence
- Very High Reflectivities
A significant presence of any of the above signatures would support a case for severity of the Bow Echo.
Considering these aspects of the Bow Echo and strong Rear Inflow Jet will help to determine the overall severity of the storm. Even stronger Bow Echo signatures should still be accompanied by some additional investigation that firm up system severity, but with the presence of a strong Rear Inflow Jet, this is one of the few signatures that can influence you directly into issuing a severe thunderstorm warning, in particular for damaging winds. Generally, radar information should never be used in isolation and should always be considered in conjunction with the near-storm environment and any reports.
Most Likely Convective Hazards
If a line of thunderstorms has been determined to be severe and contains a Bow Echo signature with a strong Rear Inflow Jet, the following convective hazards should primarily be considered for inclusion into a severe thunderstorm warning:
- Damaging winds, with some consideration to upgrading the warning to include destructive winds, especially with observed winds above 67 knots close to the surface.
See Conceptual Models for more details on why particular severe weather should be included.
References/Addendum
Houze, Robert A., M. I. Biggerstaff, S. A. Rutledge, B. F. Smull, 1989: Interpretation of Doppler Weather Radar Displays of Midlatitude Mesoscale Convective Systems. Bull. Amer. Meteor. Soc., 70, 608–619.
Weisman, M. L., 1992: The Role of Convectively Generated Rear-Inflow Jets in the Evolution of Long-Lived Mesoconvective Systems. Journal of the Atmospheric Sciences, 49, 1826-1847.